Historical Series: Photosynthesis
Unraveling Photosynthesis Step by Step:
Four Decades of Research in Theoretical and Computational Biophysics
A History in Four Parts
By Lisa Pollack
September 2012Part 2: A Task Labeled Impossible
In 1980 Schulten's formative time at the Max Planck Institute came to an end, as permanent positions there were few. He took a job 245 miles away in Bavaria, as a professor in the physics department of the Technical University of Munich. He was still eight years away from revisiting photosynthesis in his own work, but as the decade wore on he had a front row seat to some cutting edge research that would eventually bring him back to this topic.
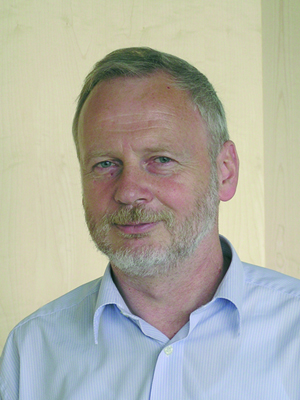
Hartmut Michel, who began a quest in 1978 to crystallize a membrane protein.
Southwest of the city center of Munich lies the town of Martinsried and in it is located the Max Planck Institute for Biochemistry. When Schulten moved to Munich in 1980 he began hearing about a fascinating project that was going on at the institute. A young biochemist there in Martinsried was trying to crystallize a membrane protein. Despite the claim in contemporary biochemistry textbooks that it was impossible to form crystals from this kind of protein, Hartmut Michel believed otherwise; an accidental observation had given him hope. Up to that point the proteins that had been crystallized were all solvated in water; since the surface of these proteins are electrically polar, they interact strongly with each other and are likely to form crystals. But membrane proteins live in lipid membranes, which are hydrophobic, and these proteins are largely nonpolar and so these membrane proteins don't interact strongly with each other.
In 1978 Hartmut Michel noticed by chance that the bacteriorhodopsin he was using in an experiment formed glass-like bodies when put in the freezer. At the time he was a post-doc in Dieter Oesterhelt's group in Würzburg, Germany, and Oesterhelt had discovered bacteriorhodopsin, an integral membrane protein, and proposed its function in the early 1970s. With the observation in the freezer, and with Oesterhelt's enthusiastic support, Michel spent the next year trying to form three-dimensional crystals of bacteriorhodopsin. While he was successful in finally obtaining the crystals, he discovered that they were not suitable for structural analysis in X-ray diffraction. Around this time Michel moved with Oesterhelt to Martinsried, where Oesterhelt became a director at the Max Planck Institute there.
But Hartmut Michel was not discouraged by his setbacks with bacteriorhodopsin. He decided to find more suitable membrane proteins and try and crystallize them instead. He chose to work with reaction center proteins from two purple bacteria, and also chlorophylls from spinach. He knew that these protein complexes were said to be part of crystalline arrays in their native environment. Using detergents, and techniques acquired from the bacteriorhodopsin work, in July 1981 Michel finally formed three-dimensional crystals of the reaction center protein of purple bacteria Rhodopseudomonas viridis. But the big test would be whether or not the reaction center crystals were of suitable quality for X-ray diffraction. In September of that year, working with Wolfram Bode, Michel found his crystals diffracted beautifully. Three years after Michel had accidentally observed crystalline-like membrane proteins in a freezer, he finally succeeded in producing high-quality crystals of a membrane protein, a task purported to be impossible. Michel points to 1981 as one of the best years of his life.
Once Hartmut Michel had his high-quality crystals, his next step was to find experts in X-ray crystallography in order to then elucidate the structure of the reaction center. Another group at the institute in Martinsried, led by Robert Huber, specialized in X-ray crystallography of proteins. In the spring of 1982 Michel gave a talk to Huber's group about his membrane protein crystallization work, seeking collaborators. Out of this seminar emerged a colleague for the project: Johann “Hans” Deisenhofer. Having joined Huber in 1971 as a PhD student, and then later converting that to a permanent position in the group, Deisenhofer did not hesitate for long to team up with Michel on the project, even though the reaction center was the largest protein at that time whose structure had yet to be determined and it was not clear that standard methods of structure determination would be suitable for the crystal.
Working at a rapid pace, and with input and support along the way from Robert Huber and Dieter Oesterhelt, the pair managed to solve the complete structure by 1985, helped by the following scientists: Kunio Miki, Otto Epp, Karl Weyer, and Heidi Gruenberg. By 1987 they had refined the structure at 2.3 Å resolution. Following their success, Hartmut Michel moved to Frankfurt in October 1987 to lead a group at the Max Planck Institute for Biophysics, and Hans Deisenhofer went to Dallas, Texas for a position at the Howard Hughes Medical Institute in March of 1988. In the fall of 1988 the two scientists, along with Robert Huber, would win the Nobel Prize in chemistry for determining the three-dimensional structure of the reaction center.
A Bonanza for a Computational Biophysicist
During his time in Munich, Schulten got to know Hartmut Michel and Hans Deisenhofer, and was always eager to hear about their progress on the reaction center over the course of the decade. In fact, Schulten soon realized that with the elucidation of the structure of a reaction center, many new calculations could be done that would have otherwise been impossible before the structure was solved. The reaction center solution had two major effects on Schulten's professional life. First, he returned to the field of photosynthesis, which had fascinated him from boyhood through graduate school. While he briefly touched on this topic in Göttingen, there he also learned about many of the major players at the forefront of photosynthesis research.
"THE REACTION CENTER SOLUTION HAD TWO MAJOR EFFECTS ON SCHULTEN'S PROFESSIONAL LIFE." |
Meanwhile, with the reaction center structure now in hand, in the late 1980s Schulten, working with Michel and Deisenhofer, began a series of molecular dynamics calculations on just a small section of the protein with his graduate student in Munich, Herbert Treutlein. They published a series of papers in 1988 on their molecular dynamics calculations. But one paper, written by Schulten, Deisenhofer and Michel, remained never to be published, a victim of fame. The referee report rejected the paper outright, claiming in this instance that one had to be super critical of high-profile scientists because otherwise the readership would easily believe everything the three famous scientists had written. Schulten is pretty sure this even happened before Michel and Deisenhofer received the Nobel Prize.
In the same year that he jump-started his return to photosynthesis by publishing computational papers that utilized the new reaction center structure, Schulten took a job at the University of Illinois at Urbana-Champaign. Soon after that, in 1989, Schulten founded the Theoretical Biophysics Group there at the Beckman Institute for Advanced Science and Technology, an interdisciplinary center at the university. Eventually the group at Beckman became known as the Theoretical and Computational Biophysics Group. It is through this group that Schulten and collaborators were able to start to piece together the many steps in photosynthesis and begin to understand it on a grand scale
Sir Anthony Leggett and Lord Rayleigh
One of the first projects Schulten embarked on at Beckman was an examination of electron transfer and its coupling to protein motion in the reaction center. This work culled from advances made by one of Schulten's colleagues, as well as one-hundred-year-old mathematics set forth by another famous physicist and Nobel Prize winner. During electron transfer in the reaction center, the electron is affected energetically by how the rest of the protein moves. The atoms in the protein vibrate due to thermal motion, and as the charges on the protein move around, the electrons exhibit slightly different quantum behavior. The reaction center protein has roughly 10,000 atoms, each vibrating in three directions, which means 30,000 vibrations. With a minimum of two parameters needed to describe the electron coupling, the result is 60,000 parameters to keep track of. To characterize this quantum system, Schulten and his graduate student Dong Xu had to dig deep.
A colleague of Schulten's in the physics department, and a next-door neighbor as well since 1988, is Sir Anthony Leggett. In 2003 Leggett would win the Nobel Prize in physics for his work explaining the theory behind superfluidity in helium-3. In 2004 Queen Elizabeth II knighted the London native for his discoveries. After his work in the 1970s that would earn him the Nobel Prize, one of the fields Leggett turned to was quantum mechanics under thermal conditions. Schulten recalls that among the many papers he published related to quantum mechanics, Leggett wrote a huge, comprehensive review article. “It was known in our physics department as the telephone book,” says Schulten, “because when it was a manuscript it was as fat as a telephone book when he sent it to the journal.”
Leggett's work on how quantum systems are affected by thermal disorder gave Dong Xu and Schulten the framework for describing how electrons in principle are coupled to the protein motion. They realized they did not explicitly need to know the 60,000 parameters, but instead could use certain functions that characterized how an electron is being affected by the vibrational motion. Additionally, Dong Xu did some detective work and hit on the mathematics derived by Lord Rayleigh in the late 1800s to describe how sound is generated; Rayleigh's equations worked out how the air, a totally disordered thermal bath, can still be imprinted with systematic coherent behavior to produce sound. “That kind of mathematics could actually be applied to the problem in the reaction center,” notes Schulten. “To understand the quantum motion of electrons, which is also coherent like sound, was the goal. The electron motions are actually being affected by the vibrations in the protein that act pretty much like the atoms in the air, statistically speaking.” Schulten is particularly proud of the elegant mathematical steps published in their papers on this topic.
After completing the electron transfer work, the mid-1990s arrived and brought an experimental collaborator from Schulten's past back into the picture. Little did Schulten realize that one inebriated evening would lead to one of the most highly cited papers in his whole career.