Morphogenesis of the Lateral Geniculate Nucleus
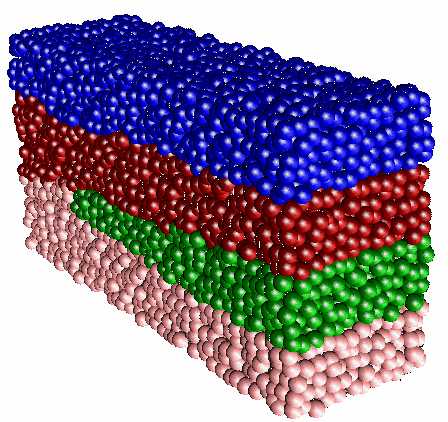
Introduction
The lateral geniculate nucleus (LGN) is a layered structure found in all mammals. It is part of the visual pathway: it receives topographically organized input from both retinae and projects to the cerebral cortex. Virtually all visual information ascends via this route. It is generally believed that at the stage of the LGN an anatomical segregation of pathways conveying form and movement signals takes place. The massive feedback from the visual cortex to the LGN suggests another LGN function, namely, selective gain modulation of cell populations in the nucleus. Based on the extensive experimental results for the cell density, cell receptive fields, and spatial arrangement of the macaque LGN layers, it is now possible to develop a detailed model for the morphogenesis (development of structure) of the macaque LGN. This work is undertaken by Svilen Tzonev, a graduate student in the Physics Department who joined our group in 1992. We collaborate closely with Professor Malpeli of the Psychology Department of the University of Illinois on this project.
Description
In primates the LGN consists of several layers of neurons separated by intervening layers of axons and dendrites. The number of distinct layers varies in different parts of the LGN. In particular, in the posterior-anterior direction (going from rear to front) the macaque LGN has regions with 6, 4, and 2 layers. The cells comprising these layers are of different types (so-called magnocellular and parvocellular cells), have different input (from ipsilateral and contralateral eyes), and have different receptive field organizations (ON and OFF types of cells, see also elsewhere in this report). Each layer maps an entire hemifield of the visual space.
It should be emphasized that the overwhelming number of studies in which neural tissue properties and developmental rules have been modeled, considered only the synaptic relationships and functional properties of the neurons. In this project we attempt to model the specific brain morphology, i.e., to explain the observed topologically complex laminar structure in three dimensions consisting of 2, 4, or 6 layers depending on the part of the LGN. The transition between the 6- and 4-layer regions occurs at the position of the optic disk (the blind spot caused by the exit of the optic nerve from the eye). With our model we want to address the issue of the role of the optic disk in this transition.
To date we have established a mathematical framework for the morphogenesis of the LGN. In this general framework, emerging receptive fields of the LGN neurons are described by a two-component vector. Each component of this vector codes for one of the cell receptive field properties: eye specificity and polarity (ON/OFF) type. Simple rules, which can be written as effective interactions between retinal terminals, govern the emergence of specific neuronal receptive fields. These rules account for the formation of a strict retinotopic map, i.e., such that neighboring parts of the retina are mapped onto neighboring parts of the LGN. As has been observed in experiments, the receptive fields of LGN neurons develop gradually, starting from one part of the nucleus. A propagating wave of development of cell receptive fields then sweeps along the main axis of the LGN. Initially, one pattern (a 6-layer one) is the most stable state of the system and, consequently, it evolves to this pattern. By means of the described local interactions this pattern has a tendency to extend throughout the whole body of the LGN. However, because of changes in the effective interaction distances between retinal terminals, at some point a 4-layer pattern becomes the preferred stable state of the system. This occurs when the ratio between the layer thickness to the effective interaction distance reaches a critical value, which has been calculated analytically. Numerical experiments confirm the value of this critical ratio.
In an artificial model, restricted to one-dimensional retinae and a two-dimensional LGN, our simulations show that the transition between the two stable patterns does indeed coincide with the location of the optic disk. The optic disk, being a perturbation, however small, causes the system to switch to its most stable state: the 4-layer pattern. The two-dimensional situation is distinctly different in a topological sense from the three-dimensional one. In two dimensions the optic disk interrupts three of the six layers completely. In three dimensions, on the other hand, the essentially one-dimensional optic disc extends its influence on a two-dimensional layer and thus its effect is significantly smaller. We are currently running computer simulations of a three-dimensional system consisting of tens of thousands of neurons. The figure illustrates a typical simulation result of the modeling in three dimensions. We are still seeking to determine the necessary conditions under which the effect of the small perturbation will lead to the transition of the 6-layer to the 4-layer pattern. The way the retinotopy constraint (the fact that neighboring parts of the retina are mapped to neighboring parts in the LGN) is included in our model appears to play a crucial role in this developmental process and requires further attention.