C&S9: Protein Folding
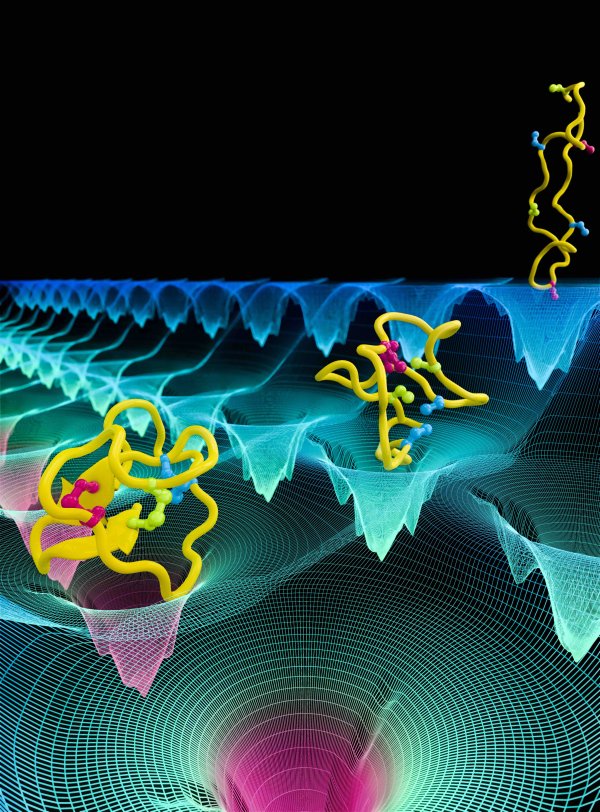
The ability of proteins to fold into their native state is essential for cell function; misfolded proteins not only lose their function, but can also cause neurodegenerative diseases, including Alzheimer and Huntington. Study of protein folding can aid in preventing protein misfolding diseases and in designing proteins with novel functions. Although most cellular proteins fold on timescales of milliseconds to seconds, the current study will focus on a 80-residue model protein named λ-repressor, which has been experimentally characterized to fold on a timescale of less than 20 μs. Our collaborator Gruebele has developed several mutants of λ-repressor that can fold on different time scales. The project will employ Center-developed long-time MD simulation with NAMD to investigate: (1) the folding pathway of λ-repressor and (2) the effect of mutations on folding of the λ-repressor.
One of the major milestones in the protein folding field was the convergence of computational and experimental timescales. Employing the Center's capability to run long time-scale simulations bridge the gap between fast folding experiments and molecular dynamics simulations, the collaboration seeks to make direct comparison between key side groups during folding as seen in experiment and simulation. Gruebele has recently developed a new experimental technique to improve the structural resolution of fast protein folding experiments, in which the formation of tertiary contacts in a protein can be detected during folding. To test out the new observations, Center researchers will accurately simulate the folding process for a duration on the order of 20 μs, which will be achieved through improved NAMD performance (TRD1) as well as through the use of the special-purpose Anton machine (D. E. Shaw research) available at the Pittsburgh Supercomputing Center. Simulating the long folding process will generate data sets that are two orders of magnitude larger than traditional MD simulation outputs. The difficulty of analyzing the huge data sets will be overcome by new developments in VMD (TRD2) such as GPU-accelerated analysis and the improved TimeLine plugin.
The study of protein folding will build upon the Center's long-standing successful collaboration with Gruebele. In particular, a complete pressure-jump refolding of λ-repressor has been characterized for the first time. Recently, Gruebele discovered variations in folding time between three mutants of λ-repressor as well as denaturation methods (high-temperature versus high-pressure). Towards the goal of a mechanistic convergence of fast folding between experiments and MD simulations, we have success- fully generated the starting structures employing NAMD for all three mutants of λ-repressor to refold in silico, mimicking the exact high-temperature or high-pressure denaturation method in vivo. The refolding of all three λ-repressor mutants are now under investigation using the special-purpose Anton machine.
The next step is to analyze the massive data set generated from λ-repressor folding trajectories. GPU-accelerated analysis and the TimeLine plugin in VMD (TRD2) will be employed to analyze and visualize the structural transitions during folding. Also, many trapped states of the protein are expected to be observed in refolding simulations based on our previous study and Markov State Models will be used to analyze the complete folding pathway. The MD simulations will not only validate the experimental approach of probing tertiary contact formation during protein folding, but also provide for the first time a quantitative one-to-one mechanistic comparison between experiment and computation at the resolution of individual structural building blocks.